Flow Control
Projects
Closed-loop Control of Low Reynolds Number Aerodynamics for Micro Air Vehicles
Team members: Tim Colonius, Mory Gharib, Sebastian Liska, Matthew Munson
Together with colleagues at the Illinois Institute of Technology and Princeton and Northeastern Universities, we are developing integrated closed-loop flow and flight control for stabilization and regulation of separated flows occurring on unmanned and micro air vehicle (UAV/MAV) wings. Inspired by the remarkable performance of birds and insects, increased lift associated with the controlled flows will lead to dramatic improvements in maneuverability, gust resistance, and a wider flight envelope. We are also studying how energy can be effectively extracted from wind gradients, in order to minimize power requirements and thereby reduce the weight of MAV. Our approach utilizes model-based, real-time control of unsteady mass injection along the wing's leading edge in order to dynamically alter the aerodynamic forces and moments, potentially eliminating traditional control surfaces whose inertia and structural limitations preclude real-time disturbance rejection algorithms. The methodology, so far demonstrated on model wings in laboratory and simulation environments, also delivers high-lift flow states that would be otherwise unstable without sensing and actuation. Specific laboratory demonstrations include the ability to dynamically cancel lift fluctuations associated with gusting flows on a time scale approaching the fast, intrinsic fluid-dynamic time scales associated with small-scale wings. A significant aspect of the work is the development of general model reduction theory and algorithms suitable for robust flow control that can be applied to a wide variety of flow control problems.
Control of Separating Flow
Team members: Beverley McKeon, Ryan Wallace
Separating flow is known to be extremely susceptible to small perturbations, a sensitivity which we utilize in this project to manipulate the geometry of the separation line and subsequently the force vector in flow over arbitrarily-shaped bodies. The ultimate goal is the demonstration of the capability of a morphing surface to provide an alternative to traditional control surfaces in a range aerospace applications, either to reduce the associated weight penalty or to expand the performance envelope. To test this capability, at present we are investigating flow over a sphere and an idealized airfoil. The sphere represents a canonical flow which has received little attention in the literature in comparison to the circular cylinder, but which has many applications, notably in sport. By introducing small surface asymmetries using a small surface roughness element and/or a morphing material, we characterize control authority over the resultant force that can be achieved on a rigidly-mounted sphere over a range of Reynolds numbers. We also consider separation on an idealized airfoil at angle of attack and at a Reynolds number appropriate for micro-air vehicle operation, performing experiments using a dynamic roughness trip to eliminate or manipulate the laminar separation bubble.
Manipulation of the Structure of Turbulent Boundary Layers
Team members: Ian Jacobi, Jeff LeHew, Beverley McKeon, Rebecca Rought
The ability to manipulate the properties of turbulent boundary layers has the potential to revolutionize a range of applications, most notably human transportation. In this project we are investigating the coupling between small surface perturbations, both static and dynamic, and wall turbulence with objectives ranging from manipulation of the spectral composition of the turbulence, the skin friction and aero-optical properties. By linking to the previously listed projects, we attempt to predict and optimize the effect of patches of surface perturbation by an extension of the input-output model framework for Navier-Stokes, with a view to designing effective manipulation and control strategies, and gaining insight into the response to static roughness, a problem of practical interest in almost all high Reynolds number flows.
Modeling and Control of Turbulent Pipe Flow
Team members: Jean-Loup Bourguignon, Beverley McKeon
The multiscale nature of turbulence, particularly at high Reynolds number, has traditionally resisted low order modeling, but the return on a successful approach would be dramatic in computation and control terms. In this project, we are developing an input-output formulation of the Navier-Stokes equation which highlights the noise amplification properties of the system in order to develop insight and models for the development of the turbulent mean velocity profile with collaborators at Caltech, Oxford and UCSB (Gayme, Doyle, Papachristodoulou and Bamieh) and the turbulent fluctuations themselves with Ati Sharma of Imperial College. While pipe flow has important applications in everyday life, it also offers geometric simplifications compared to other canonical wall flows, while maintaining aspects of expected universal behavior. The model predictions are being tested against state of the art direct numerical simulations of pipe flow, with a view to developing self-consistent, low-order representations of wall turbulence.
Morphing Surfaces for Flow Control
Team members: Ian Jacobi, Jeff LeHew, Beverley McKeon, Ryan Wallace
We investigate the coupling of small amplitude, time-dependent perturbations to surface morphology with the controlled response of fluid systems as an efficient means of actuation for flow control applications. Proofs of concept have been established in several flows and we are investigating several means to create these morphing surfaces, both on the bench and suitable for testing on an aerodynamic model in the wind tunnel. We are working with smart materials such as ionic polymer metal composite and shape memory polymer actuators capable of recreating aspects of a morphing surface, as well as mechanical proxies. This preliminary work promises to pave the way for future closed-loop control of bluff body flows and wall turbulence using bulk actuation of discrete surface regions, with the potential for a significant contribution to future aerospace design methodologies and vehicle efficiency.
Simulation, Modeling, and Control of Turbulent Jet Noise
Team members: Daniel Appelo, Tim Colonius, Matthew Inkman, Daniel Rodriguez, Aaron Towne
Since the 1950s, significant reductions in aircraft noise have been made by increasing the bypass ratio of the turbofan engine. However, the bypass ratio is reaching a practical upper bound, and significant further reductions in jet noise will require more subtle interactions with the turbulent flow field and better understanding of the mechanisms of sound generation. To date, only passive devices such as serrated nozzles have proven robust and effective enough for deployment on commercial aircraft, but recent advances in simulation and modeling promise new insights into mechanisms that can be exploited in active and, perhaps ultimately, closed-loop control. We are performing large-scale computations of turbulent jets and their radiated noise in order to provide detailed databases through which reduced-order modeling and control concepts can be evaluated. These simulations utilize new methods that permit better scalability for parallel simulations on very large numbers of processors. We are also developing reduced-order models based on Parabolized Stability Equations (PSE) and utilizing data from advanced diagnostic techniques such as large microphone arrays in order to isolate specific turbulent structures responsible for the loudest noise.
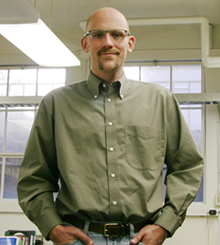
Professor Colonius develops and uses algorithms for simulation of complex, multiscale flow phenomena. Simulations can provide key insights into the mechanics of unsteady flows, including understanding of local and global instabilites, sources of sound, shock dynamics, and interactions with a disperse phase such as cavitaiton bubbles. The simulations also provide important data for reduced-order modeling efforts and control.